DOI: 10.58867/RXZI6331
The way land is planned and used in a country plays a vital role in its economic activities. This is because land use can have significant impacts on critical areas such as transport, urbanization, agriculture, and energy, among others.[1]Proper and efficient land use can support a country's sustainable development while reducing climate vulnerabilities and disasters. In contrast, inappropriate land use can cause significant issues in a warming planet, such as erosion, sedimentation, land degradation, and desertification, all of which can worsen with rising temperatures and drought risk.[2] Türkiye experienced an Environmental Kuznets curve (EKC)[3] in terms of land use transition in the last couple of decades. The balance between land use conversion to forestland (afforestation/reforestation) versus conversion of forestland to other land uses (deforestation) clearly shifted towards the latter in the early years of economic growth during this period (Figure 1).

Figure 1: The relationship between Gross Domestic Product per Capita (GDP/C) with the afforestation/reforestation (AR) and deforestation (D) rates indicates a typical EKC of a developing country for the last couple of decades in Türkiye.
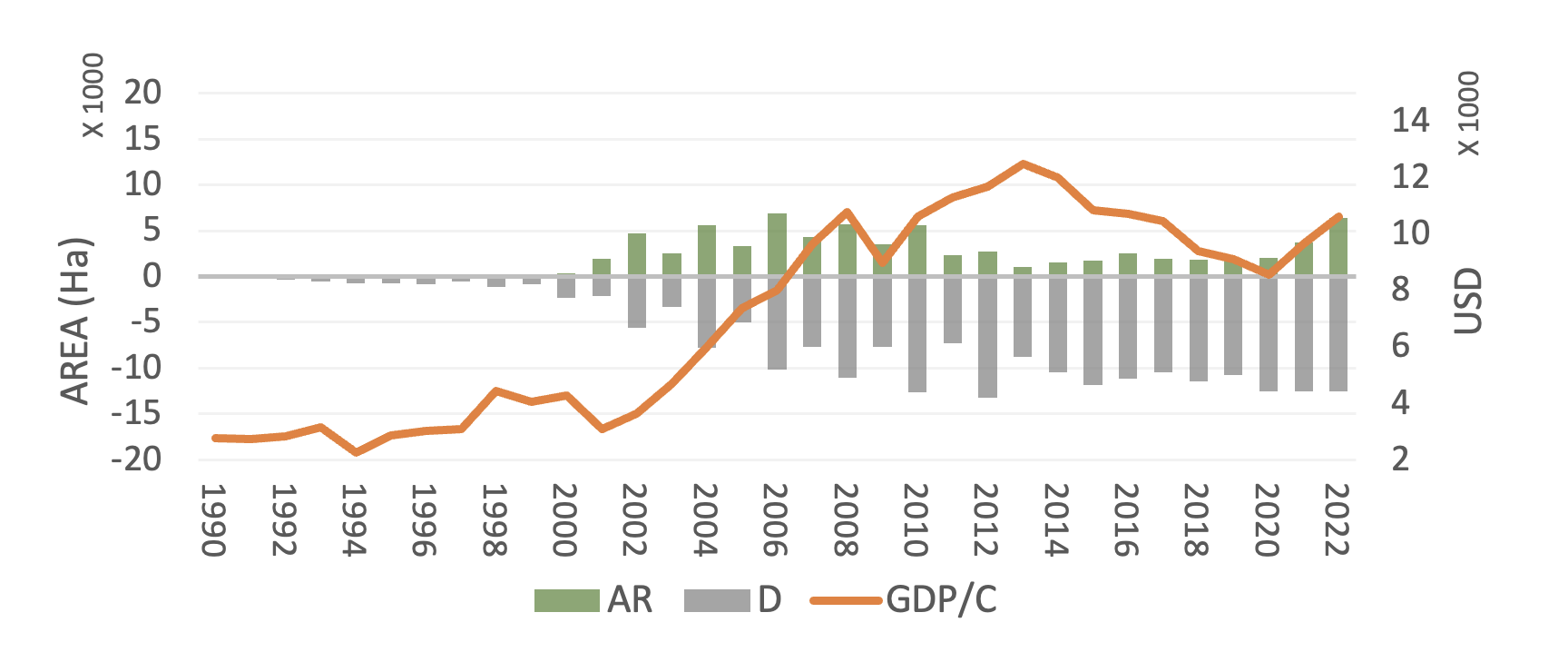
Türkiye observed a significant increase in its GDP/C from 2001 to 2008 and then a second leap following the 2009 global crisis up to 2013. During this period of economic growth, Türkiye moved from a steady neutral AR vs D state into a net D state. By the end of 2010’s, the economic growth drove some typical side effects such as urbanization, urban sprawl, and land degradation, leading to a reduction of the carbon sink capacity of the country’s land sector.[4]
In a typical EKC, the deforestation rates—a characteristic of environmental degradation— also increase during the initial phases of development. As GDP/C continues to grow, the growth becomes more environmentally friendly with rising wellbeing and public awareness. Therefore, increases in GDP/C levels subsequently become associated with net reforestation rates leading to a replenishment of forest cover (Figure 2). In the Türkiye case we do not observe a typical steady increasing GDP/C curve. The strong development until 2013 moves to an opposite direction until 2020 while the AR vs D balance continues to stay on the D side. However, an increasing trend seems to start in 2021 for both GDP/C and AR rates. From this point forward, Türkiye's economy can continue to grow with a strong environmental coupling. We assume that the improved environmental awareness and the ratification of the Paris Agreement in 2021 under the United Nations Framework Convention on Climate Change will be the driver of this upcoming sustainable development period.

Figure 2: Changes in deforestation an forest cover in time on a typical Kuznets Curve.[5]
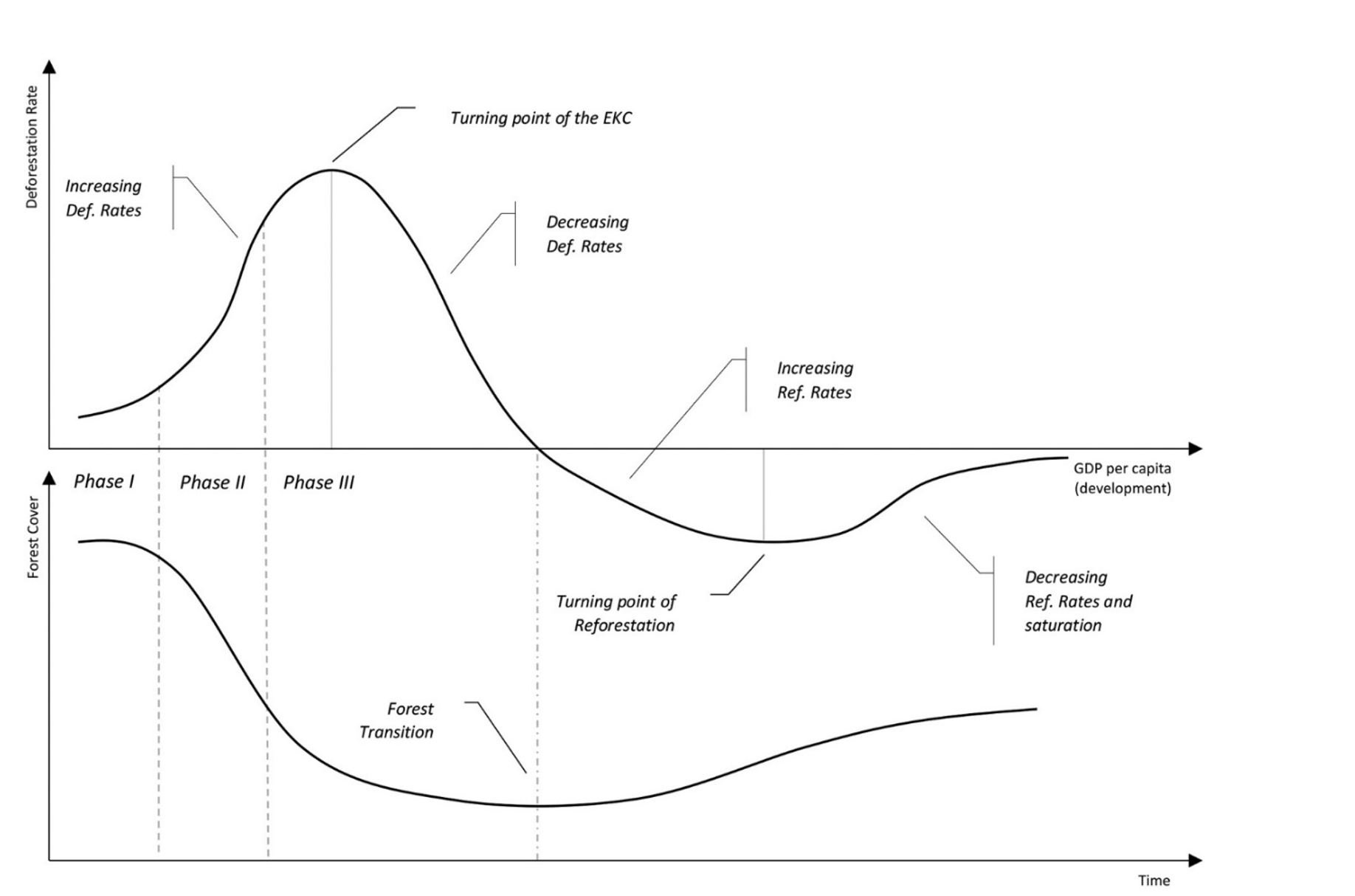
Currently, one of the critical issues related to land use globally is its potential to offset greenhouse gas emissions as a means for mitigating climate change and helping countries meet their carbon neutrality targets under the Paris Agreement.[6] Many countries have started to evaluate this aspect, and Türkiye is no exception. In this regard, this article aims to assess the potential of Türkiye's land use sector for offsetting the country’s residual greenhouse gas (GHG) emissions. By doing so, we identify the strengths and weaknesses of Türkiye's current land use practices and suggest ways to enhance them. Ultimately, this will help Türkiye achieve its climate goals and contribute to global carbon neutrality efforts.
In order to fully tap into the potential benefits of forests as carbon sinks and nature-based solutions for climate adaptation, it is crucial to prioritize the improvement of ecosystem productivity and forest cover. According to the latest data from the General Directorate of Forestry (GDF), as of 2022, forestlands reached an impressive 13,707,843 hectares equivalent to 17.5 percent of the country. However, it is worth noting that there are still vast areas of low stocked forests, covering 9,537,157 hectares.[7] By reforesting or restoring these areas, the productivity and ecological value of Türkiye's forests can be significantly increased.
According to Türkiye's latest National GHG Inventory Report,[8] the Land Use, Land Use Change and Forestry (LULUCF) sector removals account for 47.2 Mt CO2e, which offsets 8.4 percent of the total 564.4 MtCO2e GHG emissions in 2021. This represents a significant decrease from the previous NIRs, with total net removals reported at 56.9 Mt CO2e in 2020[9] and 84.0 CO2e in 2019.[10] The Forest Management (FL-FL) and Harvested Wood Products (HWP) categories are responsible for almost all of the LULUCF sector removals in Türkiye. Other categories such as Croplands, Grasslands, Settlements, and Wetlands contribute less than 1 percent of the sector total. Therefore, in order for Türkiye to achieve net zero emissions it is essential to prioritize forest and wood products management and provide additional support for other land uses that can act as carbon sinks.
The World Bank conducted an analysis to establish a projection that compares two scenarios: a High Forest Scenario (HFS) and a Business as Usual (BAU) scenario. Our HFS is a stylized scenario that showcases the full potential of the land sector by setting ambitious but achievable targets to reach carbon neutrality by 2053. This is made possible by leveraging the wide area of degraded forests available in the country. It is worth noting that the HFS scenario presented here does not involve any additional land allocation to forestland. It is a conversion of understocked forest areas (crown closure less than 10 percent) to productive forests (forest restoration).
To estimate the GHG benefits and costs of specific LULUCF activities, such as forest restoration, afforestation/reforestation, and grassland restoration, we first conducted an activity-based assessment. Our assessment revealed that A/R with fast-growing species is the most cost-effective land-based mitigation activity. However, this option is much more constrained by the availability of ecologically suitable lands with high site productivity. On the other hand, grassland restoration will not enable a large carbon sink as compared to forestry options, but it was included in the HFS due to its important co-benefits for nature and people in rural areas.
Based on our assessment, we propose that a total investment of approximately USD 9 billion can bring about the expansion of 3 million hectares of forest restoration, the establishment of 500,000 hectares of new plantations with fast-growing species, and the restoration of 1 million hectares of grasslands by 2053, the climate neutrality target year of Türkiye. To achieve this, we propose 93,750 hectares of productive forest restoration, 15,625 hectares of fast-growing plantations, and 31,250 hectares of grassland restoration per year, starting in 2023 until 2053. The table below provides a summary of the proposed activity-based mitigation options and their cumulative GHG impacts and costs. The costs have been estimated based on performance tables from the most recent GDF Activity Reports.[11]
Table 1: Activity-based mitigation options, estimated removal rates and costs
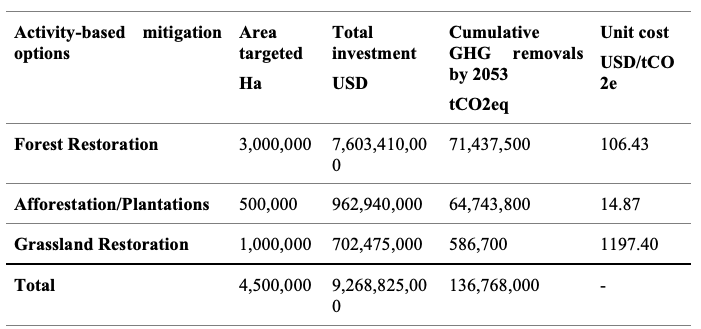
The investment amount of USD 9 billion is undeniably large, but its potential benefits in terms of ecosystem services, economic development, employment generation, and climate and disaster mitigation make it a smart investment. With the current annual budget of the GDF being around USD 1 billion, an increase of 10-20 percent in its annual budget would allow achieving these targets. Importantly, having this level of carbon sink will offset the most expensive abatement from across the economy. In other words, the most difficult and expensive emissions reductions will not need to be undertaken, saving the country many billions of dollars.
To capture the full land-based mitigation potential of the key category (FL-FL) of the LULUCF sector for the BAU and HFS scenarios up to 2053, we took into account the impacts of the above three activities along with a complementary set of sectoral Policies and Measures. Our assumptions are interconnected and have a cascading effect on the total forest carbon sink, reflecting the aggregate benefits brought by an overall improvement in land and forest management across the country’s forests. For instance, we considered that Policies and Measures designed to promote reduced harvest, minimize illegal logging, and avoid large forest fires will result in an increase in increment rates across the entire area of forest lands, which significantly enhances the total carbon sink capacity.
In the HFS scenario, we applied a harvest rate that corresponds to an annual decrease of 0.321 percent, from 22.1 million m3/yr in 2020 to 19.8 million m3/yr in 2053. Conversely, in the BAU scenario, the harvest rate followed the trend of the 1990-2019 period, with an increase from 22.02 million m3/yr in 2030, 26.38 million m3/yr of harvest in 2040, to 32.04 million m3/yr of harvest in 2053. Considering that the increment rates of the forests in Türkiye have leveled off and even started to decrease in recent years[12] the BAU scenario suggesting overharvesting seems plausible. However, the main reason for the increased harvest rate is the demand from the domestic forest industry and high timber prices. As of November 2021, the weighted average timber price was USD 149.32. Therefore, a one million m3 decrease in annual harvest would result in a loss of around 149 million USD/yr to the GDF as an opportunity cost, which may also affect the wood industry to some extent. Therefore, consultations with business and civil society will be crucial to assess trade-offs for different stakeholders.
The category of HWP is the second largest contributor to the LULUCF sector in Türkiye's GHG inventory. Our analysis assumes that the current trend in the HWP pool will persist, with sawn wood and wood panels becoming increasingly dominant over pulpwood in both the BAU and HFS scenarios. We also project a slightly faster decline in the use of fuelwood and illegal logging in the HFS compared to the BAU scenario.
In the HFS scenario, we assume that forest cover will remain stable with net zero deforestation until 2053, while in the BAU scenario forest cover is projected to decrease slightly due to factors such as urban sprawl and other drivers. It is worth noting that we did not consider the indirect positive effects of the proposed mitigation activities and policies and measures, such as decreased emissions due to reduced runoff of organic carbon or co-benefits such as increased standing volume, biodiversity benefits, erosion/sedimentation, and protection against floods, torrents, and landslides. However, when positive policies and measures are combined with additional mitigation activities, the increase in ecosystem services and total economic value could be substantial.[13]
The LULUCF sector has the potential to significantly contribute towards achieving net zero emissions by 2053 in Türkiye. However, the effectiveness of reducing GHG emissions from other sectors while fully implementing the HFS scenario is crucial for achieving this goal. For example, if the HFS is fully implemented while GHG emissions from other sectors are maintained at current levels, the LULUCF sector's contribution to carbon neutrality (offsetting emissions from other sectors) would still be about 25 percent, which is in line with the Paris Agreement (Table 2).
Table 2: Scenarios of baseline GHG emission reductions combined with LULUCF removal scenarios to assess Türkiye’s forests’ contribution to climate neutrality by 2053. The baseline year is 2019.[14]
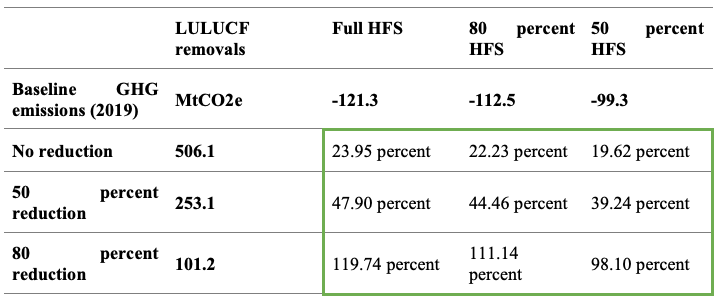
In fact, even with a 50 percent reduction in baseline GHG emissions from other sectors and full HFS implementation there will still be a large carbon debit (132.2 MtCO2 eq) which would have to be provided from carbon markets. On the other hand, if 80 percent of emission reductions in other sectors are achieved along with 50 percent of the HFS implementation, Türkiye could become almost carbon neutral with a slight debit of 3.1 MtCO2 eq. If the HFS is further implemented, Türkiye could even become a net sink with potential for carbon trading of its surplus under Article 6 of the Paris Agreement. Therefore, while reducing emissions in key emitting sectors should be the first priority for achieving carbon neutrality, an increasing carbon sink can contribute to alleviating the burden by offsetting residual emissions in hard-to-abate sectors.
Discussion and Further Considerations
In Türkiye, while the forest area[15] is increasing according to official data, the carbon sink capacity of its forests is decreasing according to the latest NIRs, which points to a reduction in the quality of the country’s forests. Therefore, there is a considerable area of land that can be restocked through afforestation or forest restoration. On the other hand, agriculture areas are decreasing due to urban sprawl around cities and migration of rural communities to urban centers (Çeler et al. 2023).[16] Restoration of degraded rangelands together with conservation agriculture may not only increase carbon stocks but also support rural development, food security, and numerous economic benefits.[17]
Our analysis assumes that Türkiye initiated a HFS by 2023. Any delay in applying the HFS may raise the cost or lower the level of carbon sink. It is important to note that the HFS presented in our analysis is not an exact estimate; it is rather meant to indicate the potential contribution of the LULUCF sector to Türkiye’s carbon neutrality in the next three decades. The forest land category is the largest and only carbon sink mechanism in the country. Therefore, it is crucial to fully mobilize its potential. This comes with co-benefits such as reducing the urban heat island effect, flood and landslide control, erosion reduction, groundwater recharge, and biodiversity conservation, among others.[18]
It is worth noting that there is potential for additional land-based GHG removals in Türkiye. According to the Ministry of Agriculture and Forestry there are approximately 2 million hectares of abandoned farmlands in rural areas due to urban migration. If the government of Türkiye develops a policy to convert these lands into productive orchards or fast-growing plantations, there can be additional carbon benefits.
The wetlands and settlement LULUCF categories have not been directly discussed in this article as GHG estimates for these categories are not well established in Türkiye’s NIR. However, conversion from forests to these categories are assessed under deforestation. In the coming decades, we can expect a lower rate of reservoir construction compared to the last few decades, since the hydroelectric potential of the country has been almost fully mobilized.[19] On the other hand, settlements can further expand (urban sprawl). This may cause carbon stocks to decrease around cities but can be slowed with smart land use solutions. Urban development can even increase carbon stocks, especially around semiarid cities if there is water for irrigation available.
Conclusions
Türkiye’s GHG removals in the LULUCF sector currently offset around 8.4 percent of its total GHG emissions according to the latest NIR.[20] To increase CO2e removals from the LULUCF sector to enable net zero emissions by 2053 Türkiye must focus on the following policies and measures:
-
Achieve net zero deforestation by 2025;
-
The increment rate of standing forests should be enhanced to further increase the growing stock of the country’s forests. This can be achieved through reduced harvesting and improved forest management approaches, keeping the harvest rate around 20 million m3/yr during the 2023-2053 period,
-
3 million hectares of degraded forests should be rehabilitated in the period 2023-2053,
-
500,000 hectares of land suitable for A/R should be allocated for fast growing species plantations during the period of 2023-2053,
-
Additional actions including grassland rehabilitation, climate smart agriculture practices and decreased illegal logging should be pursued.
These land-based activities and complementary Policies and Measures can enable LULUCF removals to reach well over 100 million tCO2eq/yr by 2053, the year in which Türkiye is plans to reach carbon neutrality.
There is a significant potential for the land sector to increase its GHG removals and further offset residual emissions from the other sectors of the economy that are harder and more expensive to abate on the road to Türkiye’s carbon neutrality.
[1] Jianbao Li, Xianjin Huang, Xiaowei Chuai, and Hong Yang, “The Impact of Land Urbanization on Carbon Dioxide Emissions in the Yangtze River Delta, China: A Multiscale Perspective,” Cities, Vol. 116 (2021): 103275. doi: 10.1016/J.CITIES.2021.103275; Asif Raihan, “The Dynamic Nexus between Economic Growth, Renewable Energy Use, Urbanization, Industrialization, Tourism, Agricultural Productivity, Forest Area, and Carbon Dioxide Emissions in the Philippines,” Energy Nexus, Vol. 9 (2023): 100180. doi: 10.1016/J.NEXUS.2023.100180
[2] Donia Jendoubi, Md Sarwar Hossain, Markus Giger, Jelena Tomicevic-Dubljevic, Mohamed Ouessar, Hanspeter Liniger, and Chinwe Ifejika Speranza, “Local Livelihoods and Land Users’ Perceptions of Land Degradation in Northwest Tunisia,” Environmental Development, Vol. 33 (2020): 100507. doi: 10.1016/J.ENVDEV.2020.100507; Gebeyehu Taye, Tesfaye Teklesilassie, Daniel Teka, and Henok Kassa, “Assessment of Soil Erosion Hazard and Its Relation to Land Use Land Cover Changes: Case Study from Alage Watershed, Central Rift Valley of Ethiopia,” Heliyon, Vol. 9, No. 8 (2023):e18648. doi: 10.1016/J.HELIYON.2023.E18648
[3] Nkwetta Ajong Aquilas, Alfred Kechia Mukong, Jude Ndzifon Kimengsi, and Forbe Hodu Ngangnchi. “Economic Activities and Deforestation in the Congo Basin: An Environmental Kuznets Curve Framework Analysis,” Environmental Challenges, Vol. 8 (2022): 100553. doi: 10.1016/J.ENVC.2022.100553; Patrícia Hipólito Leal and António Cardoso Marques, “The Evolution of the Environmental Kuznets Curve Hypothesis Assessment: A Literature Review under a Critical Analysis Perspective,” Heliyon, Vol. 8 No. 11 (2022): :e11521. doi: 10.1016/J.HELIYON.2022.E11521
[5] Nicola Caravaggio, “Economic Growth and the Forest Development Path: A Theoretical Re-Assessment of the Environmental Kuznets Curve for Deforestation,” Forest Policy and Economics, Vol. 118 (2020) :102259. doi: 10.1016/J.FORPOL.2020.102259
[6] Jingyi Liu, Qianqian Yan, and Menghan Zhang, “Ecosystem Carbon Storage Considering Combined Environmental and Land-Use Changes in the Future and Pathways to Carbon Neutrality in Developed Regions,” Science of The Total Environment, Vol. 903 (2023): 166204. doi: 10.1016/J.SCITOTENV.2023.166204; Antti Majava, Tere Vadén, Tero Toivanen, Paavo Järvensivu, Ville Lähde, and Jussi T. Eronen, “Sectoral Low-Carbon Roadmaps and the Role of Forest Biomass in Finland’s Carbon Neutrality 2035 Target,” Energy Strategy Reviews, Vol. 41 (2022): 100836. doi: 10.1016/J.ESR.2022.100836; S. L. Swamy, H. Darro, A. Mishra, Rattan Lal, Amit Kumar, and Tarun Kumar Thakur, “Carbon Stock Dynamics in a Disturbed Tropical Forest Ecosystem of Central India: Strategies for Achieving Carbon Neutrality,” Ecological Indicators, Vol. 154 (2023): 110775. doi: 10.1016/J.ECOLIND.2023.110775
[13] A. Weatherall et al., “Defining Climate-Smart Forestry,” in: R. Tognetti, Smith, M., Panzacchi, P. (eds) Climate-Smart Forestry in Mountain Regions. Managing Forest Ecosystems, Vol 40 (Springer, Cham, 2020). https://doi.org/10.1007/978-3-030-80767-2_2
[15] The forest area increase reported by the GDF each year in its Activity Reports represents the area covering both stocked and unstocked lands allocated for forest land use. The term “stocked” refers to a forest crown closure of over 10 percent and “unstocked” below 10 percent. Some of these lands can be afforested or restored in time provided they can still support a woody vegetation, which is nto the case for a large portion of these lands.
[16] Erda Çeler, Yusuf Serengil and Ufuk Özkan, “A Comparative Assessment of Forest/Green Cover and the Awareness of Forestry District Managers,” Environmental Monitoring and Assessment, Vol. 195, No. 520 (2023): 520.
[17] N. Stoeckl, T. Chaiechi, M. Farr, D. Jarvis, J. G. Álvarez-Romero, M. J. Kennard, V. Hermoso, and R. L. Pressey, “Co-Benefits and Trade-Offs between Agriculture and Conservation: A Case Study in Northern Australia,” Biological Conservation, Vol. 191 (2015): 478–94. doi: 10.1016/J.BIOCON.2015.07.032
[18] Benício Arrais, Roberta Maria, Karina Vieiralves Linhares, Maria Amanda Nobre Lisboa, Gabriel Venâncio Cruz, Leonardo Vitor Alves da Silva, Arthur da Silva Nascimento, Maria Arlene Pessoa da Silva, Leonardo Silvestre Gomes Rocha, Marcos Antônio Drumond, Rafael Gonçalves Tonucci, and João Tavares Calixto Júnior. “Carbon Stock and Sequestration as a Form of Payment for Environmental Services in a Sedimentary Basin Humid Forest Refuge in Brazilian Semiarid,” Environmental Development, Vol. 45 (2023) :100796. doi: 10.1016/J.ENVDEV.2022.100796; Elivane Salete Capellesso, Anamaria Cequinel, Renato Marques, Tanise Luisa Sausen, Cimélio Bayer, and Marcia Cristina Mendes Marques, “Co-Benefits in Biodiversity Conservation and Carbon Stock during Forest Regeneration in a Preserved Tropical Landscape,” Forest Ecology and Management, Vol. 492 (2021): 119222. doi: 10.1016/J.FORECO.2021.119222
[19] Mehmet Bilgili, Harun Bilirgen, Arif Ozbek, Firat Ekinci, and Tugce Demirdelen. “The Role of Hydropower Installations for Sustainable Energy Development in Turkey and the World,” Renewable Energy, Vol. 126 (2018): 755–64. doi: 10.1016/J.RENENE.2018.03.089